Biofuel (also called agrofuel) is a basic abbreviation of biorganic fuel. This is a scientific name for any plant or animal substance that can burn (combustible (fuel) organism (organic) of two types (bi-), plant and animal). Biofuel is an alternative considered to replace petroleum gas (gasoline or petrol). Most transportation vehicles require high power density provided by internal combustion engines. These engines require clean burning fuels, which are generally in liquid form, and to a lesser extent, compressed gaseous phase. Liquids are more portable because they have high energy density, and they can be pumped, which makes handling easier. This is why most transportation fuels are liquids.
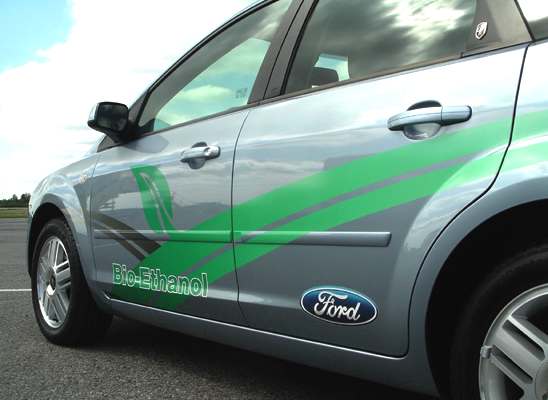
Ford
Focus Bio Ethanol car
UK
Biofuel consumption
2005
- 118 million litres
2006
- 264 million litres
2007
- 500 million litres
Source:
HM Revenue & Custom
Average
UK unleaded petrol prices
Feb
2008 - 104 pence per litre
Feb
2007- 86 pence per litre
Feb
2006 - 90pence per litre
Feb
2005 - 80pence per litre
Feb
2004 - 81.9pence per litre
Feb
2003 - 80.2pence per litre
Non-transportation applications can usually tolerate the low power-density of external combustion engines, that can run directly on less-expensive solid biomass fuel, for combined heat and power. One type of biomass is wood, which has been used for millennia in varying quantities, and more recently is finding increased use. Two billion people currently cook every day, and heat their homes in the winter by burning biomass, which is a contributor to man-made climate change
global warming. The black soot that is being carried from Asia to polar ice caps is causing them to melt faster in the summer. In the 19th century, wood-fired steam engines were common, contributing significantly to industrial revolution unhealthy air pollution. Coal is a form of biomass that has been compressed over millennia to produce a non-renewable, highly-polluting
fossil fuel.
Wood and its byproducts can now be converted into biofuels such as woodgas, methanol or ethanol fuel.
Biofuel is considered by some as a means of reducing greenhouse gas emissions and increasing energy security by providing an alternative to fossil
fuels. However, In October 2007, Nobel Laureate Paul Crutzen published findings that the release of Nitrous Oxide (N2O) from rapeseed oil, and corn (maize), contribute more to global warming than the fossil fuels they replace. However, the Crutzen paper goes on to say that crops with less nitrogen demand, such as grasses and woody coppicing will have positive but lower climate
impacts. In February 2008, two articles were published in Science concluding that clearing land for biofuel production produce twice as much greenhouse gas than the U.N. IPCC had previously estimated.
Biofuels are used globally. Biofuel industries are expanding in Europe, Asia and the Americas. The most common use for biofuels is automotive transport (for example E10 fuel). Increased American and European demand has led to clearing land for Palm Oil plantations. Locations such as Indonesia are subject to deforestation and the accompanying displacement of indigenous peoples. In some areas use of pesticides for biofuel crops are disrupting clean water
supplies.
Biofuel can be theoretically produced from any (biological) carbon source. The most common by far is photosynthetic plants that capture solar energy. Many different plants and plant-derived materials are used for biofuel manufacture.
The greatest technical challenge is to develop ways to convert biomass energy specifically to liquid fuels. To achieve this, the two most common strategies are:
To grow sugar crops (sugar cane, and sugar beet), or starch (corn/maize), and then use yeast fermentation to produce ethanol (ethyl alcohol).
To grow plants that (naturally) produce oils, such as algae, or jatropha. When these oils are heated, their viscosity is reduced, and they can be burned directly in a diesel engine. The oils can also be chemically processed to produce
biodiesel.
History and Policy
Humans have used biomass fuels in the form of solid biofuels for heating and cooking since the discovery of fire. Following the discovery of electricity, it became possible to use biofuels to generate electrical power as well. However, the discovery and use of fossil fuels: coal, gas and oil, have dramatically reduced the amount of biomass fuel used in the developed world for transport, heat and power.
Liquid biofuels have been used since the early days of the automobile industry. Nikolaus August Otto, the German inventor of the internal combustion engine, conceived his invention to run on ethanol.[citation needed] Rudolf Diesel, the German inventor of the Diesel engine, designed it to run on peanut oil, and Henry Ford originally designed the Ford Model T, a car produced from 1903 to 1926, to run completely on hemp derived biofuel. However, when large supplies of crude oil were discovered in Pennsylvania and Texas, petroleum based fuels became inexpensive, and soon were widely used. Cars and trucks began using fuels derived from mineral oil/petroleum: gasoline/petrol or
diesel.
Nevertheless, before World War II, and during the high demand wartime period, biofuels were valued as a strategic alternative to imported oil. Wartime Germany experienced extreme oil shortages, and many energy innovations resulted. This include the powering of some of its vehicles using a blend of gasoline with alcohol fermented from potatoes, called
Reichskraftsprit. In Britain, grain alcohol was blended with petrol by the Distillers Company Limited under the name Discol and marketed through Esso's affiliate
Cleveland.
During the peacetime post-war period, inexpensive oil from the Middle East contributed in part to the lessened economic and geopolitical interest in biofuels. Then in 1973 and 1979, geopolitical conflict in the Middle East caused
OPEC to cut exports, and non-OPEC nations experienced a very large decrease in their oil supply. This "energy crisis" resulted in severe shortages, and a sharp increase in high demand oil-based products, notably petrol/gasoline. There was also increased interest from governments and academics in energy issues and biofuels. Throughout history, the fluctuations of supply and demand, energy policy, military conflict, and the environmental impacts, have all contributed to a highly complex and volatile market for energy and fuel.
In the year 2000 and beyond, renewed interest in biofuels has been seen. The drivers for biofuel research and development include rising oil prices, concerns over the potential oil peak,
greenhouse gas emissions (causing global warming and climate change), rural development interests, and instability in the Middle East.
Biomass
Biomass is material derived from recently living organisms. This includes plants, animals and their by-products. For example, manure, garden waste and crop residues are all sources of biomass. It is a renewable energy source based on the carbon cycle, unlike other natural resources such as petroleum, coal, and nuclear fuels. Agricultural products specifically grown for biofuel production include corn, switchgrass, and soybeans, primarily in the United States; rapeseed, wheat and sugar beet primarily in Europe; sugar cane in Brazil; palm oil and miscanthus in South-East Asia; sorghum and cassava in China; and jatropha in India. Hemp has also been proven to work as a biofuel. Biodegradable outputs from industry, agriculture, forestry and households can be used for biofuel production, either using anaerobic digestion to produce biogas, or using second generation biofuels; examples include straw, timber, manure, rice husks, sewage, and food waste. The use of biomass fuels can therefore contribute to waste management as well as fuel security and help to prevent climate change, though alone they are not a comprehensive solution to these problems.
Bioenergy from waste
Using waste biomass to produce energy can reduce the use of fossil fuels, reduce greenhouse gas emissions and reduce pollution and waste management problems. A recent publication by the European Union highlighted the potential for waste-derived bioenergy to contribute to the reduction of global warming. The report concluded that 19 million tons of oil equivalent is available from biomass by 2020, 46% from bio-wastes: municipal solid waste (MSW), agricultural residues, farm waste and other biodegradable waste
streams.
Landfill sites generate gases as the waste buried in them undergoes anaerobic digestion. These gases are known collectively as landfill gas. This can be burned and is considered a source of renewable energy, even though landfill disposal are often non-sustainable. [Landfill gas (LFG)] can be burned either directly for heat or to generate electricity for public consumption. Landfill gas contains approximately 50 percent methane, the same gas that is found in natural
gas.
If landfill gas is not harvested, it escapes into the atmosphere: this is not desirable because methane is a greenhouse gas, with more global warming potential than carbon
dioxide. Over a time span of 100 years, methane has a global warming potential of 23 relative to
CO2. Therefore, during this time, one ton of methane produces the same greenhouse gas (GHG) effect as 23 tons of CO2.[citation needed] When methane burns the formula is CH4 + 2O2 = CO2 + 2H2O So by harvesting and burning landfill gas, its global warming potential is reduced a factor of 23, in addition to providing energy for heat and power.
Frank Keppler and Thomas Rockmann discovered that living plants also produce methane
CH4. The amount of methane produced by living plants is 10 to 100 times greater than that produced by dead plants (in an aerobic
environment) but does not increase global warming because of the carbon
cycle.
Anaerobic digestion can be used as a distinct waste management strategy to reduce the amount of waste sent to landfill and generate methane, or biogas. Any form of biomass can be used in anaerobic digestion and will break down to produce methane, which can be harvested and burned to generate heat, power or to power certain automotive vehicles.
A 3 MW landfill power plant would power 1,900 homes. It would eliminate 6,000 tons per year of methane from getting into the
environment. It would eliminate 18,000 tons per year of CO2 from fossil fuel
replacement. This is the same as removing 25,000 cars from the road, or planting 36,000 acres (146 km²) of
forest, or not using 305,000 barrels of oil per year.
First generation biofuels
'First-generation fuels' refer to biofuels made from sugar, starch, vegetable oil, or animal fats using conventional
technology.
The most common first generation biofuels are listed below.
Sugar
beet
Vegetable oil
Vegetable oil can be used for either food or fuel; the quality of the oil may be lower for fuel use. Vegetable oil can be used in many older diesel engines (equipped with indirect injection systems), but only in warm climates. In most cases, vegetable oil is used to manufacture biodiesel, which is compatible with most diesel engines when blended with conventional diesel fuel. MAN B&W Diesel, Wartsila and Deutz AG offer engines that are compatible with straight vegetable oil. Used vegetable oil is increasingly being processed into biodiesel, and at a smaller scale, cleaned of water and particulates and used as a fuel.
Biodiesel
Biodiesel is the most common biofuel in Europe. It is produced from oils or fats using transesterification and is a liquid similar in composition to mineral diesel. Its chemical name is fatty acid methyl (or ethyl) ester (FAME). Oils are mixed with sodium hydroxide and methanol (or ethanol) and the chemical reaction produces biodiesel (FAME) and glycerol. 1 part glycerol is produced for every 10 parts biodiesel.
Biodiesel can be used in any diesel engine when mixed with mineral diesel. In some countries manufacturers cover their diesel engines under warranty for 100% biodiesel use, although Volkswagen Germany, for example, asks drivers to make a telephone check with the VW environmental services department before switching to 100% biodiesel (see biodiesel use). Many people have run their vehicles on biodiesel without problems. However, the majority of vehicle manufacturers limit their recommendations to 15% biodiesel blended with mineral diesel. In many European countries, a 5% biodiesel blend is widely used and is available at thousands of gas
stations.
In the USA, more than 80% of commercial trucks and city buses run on diesel. Therefore "the nascent U.S. market for biodiesel is growing at a staggering rate—from 25 million gallons per year in 2004 to 78 million gallons by the beginning of 2005. By the end of 2006 biodiesel production was estimated to increase fourfold to more than 1 billion gallons," energy expert Will Thurmond writes in an article for the July-August 2007 issue of THE FUTURIST magazine.
Bioalcohols
Biologically produced alcohols, most commonly ethanol, and less commonly propanol and butanol, are produced by the action of microorganisms and enzymes through fermentation of sugars or starches (easiest), or celulose (which is more difficult). Biobutanol (also called biogasoline) is often claimed to provide a direct replacement for gasoline, because it can be used directly in a gasoline engine (in a similar way to biodiesel in diesel
engines).
Butanol is formed by ABE fermentation (acetone, butanol, ethanol) and experimental modifications of the process show potentially high net energy gains with butanol as the only liquid product. Butanol will produce more energy and allegedly can be burned "straight" in existing gasoline engines (without modification to the engine or
car), and is less corrosive and less water soluble than ethanol, and could be distributed via existing infrastructures. DuPont and BP are working together to help develop Butanol.
Ethanol fuel is the most common biofuel worldwide, particularly ethanol fuel in Brazil. Alcohol fuels are produced by fermentation of sugars derived from wheat, corn, sugar beets, sugar cane, molasses and any sugar or starch that alcoholic beverages can be made from (like potato and fruit waste, etc.). The ethanol production methods used are enzyme digestion (to release sugars from stored starches, fermentation of the sugars, distillation and drying. The process requires significant energy input for heat (often unsustainable natural gas fossil fuel).
Cellulosic ethanol production uses non food crops or inedible waste products, which has less of an impact on food. Lignocellulose is the "woody" structural material of plants. This feedstock is abundant and diverse, and in some cases (like citrus peels or sawdust) it is a significant industry-specific disposal problem.
Producing ethanol from cellulose is a more difficult-and-expensive additional-step technical problem to solve. Ruminant livestock (like
cattle) eat grass and then use slow enzymatic digestive processes to break it into glucose
(sugar). In cellulosic ethanol laboratories, various experimental processes are being used to do the same thing, and then do the above process to make ethanol fuel.
Some scientists have expressed concern that if experimental recombinant DNA genetic engineering continues to be used to develop unprecedented enzymes that break down wood much faster than in nature, such microscopic life forms may accidentally be released into nature, grow exponentially, be distributed by the wind, and eventually destroy the structure of all trees, ending all Earthly life that breathes oxygen released by photosynthesis in
trees.
Ethanol can be used in petrol engines as a replacement for gasoline; it can be mixed with gasoline to any percentage. Most existing automobile petrol engines can run on blends of up to 15% bioethanol with petroleum/gasoline. Gasoline with ethanol added has higher octane, which means that your engine can typically burn hotter and more efficiently. In high altitude (thin air) locations, some states mandate a mix of gasoline and ethanol as a winter oxidizer to reduce atmospheric
pollution emissions.
Ethanol fuel has less BTU energy content, which means it takes more fuel (volume and mass) to go the same distance. More-expensive premium fuels contain less, or no, ethanol. In high-compression engines, less ethanol, slower-burning premium fuel is required to avoid harmful pre-ignition (knocking). Very-expensive aviation gasoline (Avgas) is 100 octane made from 100% petroleum. The high price of zero-ethanol Avgas does not include federal-and-state road-use taxes.
Ethanol is very corrosive to fuel systems, rubber hoses-and-gaskets, aluminum, and combustion chambers. It is therefore illegal to use fuels containing alcohol in aircraft. Ethanol is incompatible with marine fiberglass fuel tanks (it makes them leak). For higher ethanol percentage blends, and 100% ethanol vehicles, engine modifications are required.
Corrosive ethanol cannot be transported in petroleum pipelines, so more-expensive over-the-road stainless-steel tank trucks increase the cost and energy consumption required to deliver ethanol to the customer at the pump.
When considering the total energy consumed by farm equipment, cultivation, planting, fertilizers, pesticides, herbicides, and fungicides made from petroleum, irrigation systems, harvesting, transport of feedstock to processing plants, fermentation, distillation, drying, transport to fuel terminals and retail pumps, and lower ethanol fuel energy content, the net energy content value added and delivered to consumers is very small. And, the net benefit (all things considered) does little to reduce un-sustainable imported oil and fossil fuels required to produce the
ethanol.
Many car manufacturers are now producing flexible-fuel vehicles (FFV's), which can safely run on any combination of bioethanol and petrol, up to 100% bioethanol. They dynamically sense exhaust oxygen content, and adjust the engine's computer systems, spark, and fuel injection accordingly. This adds initial cost and ongoing increased vehicle maintenance. Efficiency falls and pollution emissions increase when FFV system maintenance is needed (regardless of the 0%-to-100% ethanol mix being used), but not performed (as with all vehicles). FFV internal combustion engines are becoming increasingly complex, as are multiple-propulsion-system FFV hybrid vehicles, which impacts cost, maintenance, reliability, and useful lifetime longevity.
Alcohol mixes with both petroleum and with water, so ethanol fuels are often diluted after the drying process by absorbing environmental moisture from the atmosphere. Water in alcohol-mix fuels reduces efficiency, makes engines harder to start, causes intermittent operation (sputtering), and oxidizes
aluminum (carburetors) and steel components (rust).
Even dry ethanol has roughly one-third lower energy content per unit of volume compared to gasoline, so larger / heavier fuel tanks are required to travel the same distance, or more fuel stops are required. With large current un-sustainable, non-scalable subsidies, ethanol fuel still costs much more per unit of distance traveled than current high gasoline
prices.
Methanol is currently produced from natural gas, a non-renewable fossil fuel. It can also be produced from biomass as biomethanol. The methanol economy is an interesting alternative to the hydrogen economy, compared to today's hydrogen produced from natural gas, but not
hydrogen production directly from water and state-of-the-art clean solar thermal energy
processes.
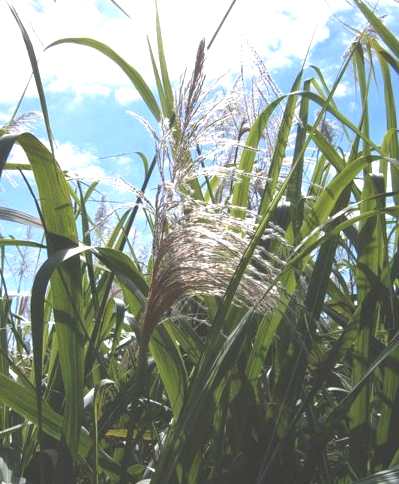
Sugar
cane flowering in Australia
BioGas
Biogas is produced by the process of anaerobic digestion of organic material by anaerobes. It can be produced either from biodegradable waste materials or by the use of energy crops fed into anaerobic digesters to supplement gas yields. The solid byproduct, digestate, can be used as a biofuel or a fertilizer.
Biogas contains methane and can be recovered from industrial anaerobic digesters and mechanical biological treatment systems. Landfill gas is a less clean form of biogas which is produced in landfills through naturally occurring anaerobic digestion. If it escapes into the atmosphere it is a potent greenhouse gas.
Oils and gases can be produced from various biological wastes:
Thermal depolymerization of waste can extract methane and other oils similar to petroleum.
GreenFuel Technologies Corporation developed a patented bioreactor system that uses nontoxic photosynthetic algae to take in smokestacks flue gases and produce biofuels such as biodiesel, biogas and a dry fuel comparable to
coal.
Solid Biofuels: Examples include wood, charcoal, and dried manure.
Syngas
Syngas is produced by the combined processes of pyrolysis, combustion, and gasification. Biofuel is converted into carbon monoxide and energy by pyrolysis. A limited supply of oxygen is introduced to support combustion. Gasification converts further organic material to hydrogen and additional carbon monoxide.
The resulting gas mixture, syngas, is itself a fuel. Using the syngas is more efficient than direct combustion of the original biofuel; more of the energy contained in the fuel is extracted.
Syngas may be burned directly in internal combustion engines. The wood gas generator is a wood-fueled gasification reactor mounted on an internal combustion engine. Syngas can be used to produce methanol and hydrogen, or converted via the Fischer-Tropsch process to produce a synthetic petroleum substitute. Gasification normally relies on temperatures >700°C. Lower temperature gasification is desirable when co-producing biochar.
Second generation biofuels
Supporters of biofuels claim that a more viable solution is to increase political and industrial support for, and rapidity of, second-generation biofuel implementation from non food crops, including cellulosic biofuels.[20] Second-generation biofuel production processes can use a variety of non food crops. These include waste biomass, the stalks of wheat, corn, wood, and special-energy-or-biomass crops (e.g. Miscanthus). Second generation (2G) biofuels use biomass to liquid technology, including cellulosic biofuels from non food
crops. Many second generation biofuels are under development such as biohydrogen, biomethanol, DMF, Bio-DME, Fischer-Tropsch diesel, biohydrogen diesel, mixed alcohols and wood diesel.
Third generation biofuels
Algae fuel, also called oilgae or third generation biofuel, is a biofuel from algae. Algae are low-input/high-yield (30 times more energy per acre than land) feedstocks to produce
biofuels and algae fuel are biodegradable:
With the higher prices of oil, there is much interest in algaculture (farming algae).
One advantage of many biofuels over most other fuel types is that they are biodegradable, and so relatively harmless to the environment if
spilled.
The United States Department of Energy estimates that if algae fuel replaced all the petroleum fuel in the United States, it would require 15,000 square miles (38,849 square kilometers), which is a few thousand miles larger than
Maryland.
Efforts and promotion
Recognizing the importance of implementing bioenergy, there are international organizations such as IEA Bioenergy, established in 1978 by the OECD International Energy Agency (IEA), with the aim of improving cooperation and information exchange between countries that have national programs in bioenergy research, development and deployment. The U.N. International Biofuels Forum is formed by Brazil, China, India, South Africa, the United States and the European
Commission. The world leaders in biofuel development and use are Brazil, United States, France, Sweden and
Germany.
Brazil
In Brazil, the government hopes to build on the success of the Proálcool ethanol program by expanding the production of biodiesel which must contain 2% biodiesel by 2008, increasing to 5% by 2013.
USA
A senior member of the House Energy and Commerce Committee Congressman Fred Upton has introduced legislation to use at least E10 fuel by 2012 in all cars in the USA.
General Motors is starting a project to produce E85 fuel from cellulose ethanol for a projected cost of
$1 a gallon. The raw materials can be as simple as corn stalks and scrap petroleum-based vehicle
tires, but used tires are an expensive feedstock with other more-valuable uses. GM has over 4 million E85 cars on the road now and by 2012 half of the production for the U.S. will run on E85 fuel. Coskata Inc. is building two new plants for the ethanol fuel. The process is five times more energy efficient than corn based ethanol.
The greenhouse gas emissions are reduced by 86% for cellulose compared to corn’s 29%
reduction.
In 2006, the United States president George W. Bush said in a State of the Union speech that the US is "addicted to oil" and should replace 75% of imported oil by 2025 by alternative sources of energy including biofuels.
The 2007-12-19 U.S. Energy Independence and Security Act of 2007 requires American “fuel producers to use at least 36 billion gallons of biofuel in 2022. This is nearly a fivefold increase over current levels.”[29] This is causing a significant agricultural resource shift away from food production to biofuels. American food exports have decreased (increasing grain prices worldwide), and US food imports have increased significantly.
Most biofuels are not currently cost-effective without significant subsidies. "America's ethanol programme is a product of government subsidies. There are more than 200 different kinds, as well as a 54 cents-a-gallon tariff on imported ethanol. That keeps out greener Brazilian ethanol, which is made from sugar rather than maize. Federal subsidies alone cost $7 billion a year (equal to around $1.90 a
gallon)."
Europe
The European Union in its biofuels directive (updated 2006) has set the goal that for 2010 that each member state should achieve at least 5.75% biofuel usage of all used traffic fuel. By 2020 the figure should be 10%. As of January 2008 these aims are being reconsidered in light of certain environmental and social concerns associated with biofuels such as rising food prices and
deforestation.
France is the second largest biofuel consumer among the EU States in 2006. According to the Ministry of Industry, France's consumption increased by 62.7% to reach 682,000 toe (i.e. 1.6% of French fuel consumption). Biodiesel represents the largest share of this (78%, far ahead of bioethanol with 22%). The unquestionable biodiesel leader in Europe is the French company Diester Industrie. In bioethanol, the French agro-industrial group Téréos is increasing its production capacities. Germany itself remained the largest European biofuel consumer, with a consumption estimate of 2.8 million tons of biodiesel (equivalent to 2,408,000 toe), 0.71 million ton of vegetable oil (628.492 toe) and 0.48 million ton of bioethanol (307,200 toe).
The biggest biodiesel German company is ADM Oelmühle Hamburg AG, which is a subsidiary of the American group Archer Daniels Midland Company. Among the other large German producers, MUW (Mitteldeutsche Umesterungswerke GmbH & Co KG) and EOP Biodiesel AG. A major contender in terms of bioethanol production is the German sugar corporation, Südzucker.
The Spanish group Abengoa, via its American subsidiary Abengoa Bioenergy, is the European leader in production of bioethanol.
In the United Kingdom the Renewable Transport Fuel Obligation (RTFO) (announced 2005) is the requirement that by 2010 5% of all road vehicle fuel is renewable. In 2008 a critical report by the Royal Society stated that biofuels risk failing to deliver significant reductions in greenhouse gas emissions from transport and could even be environmentally damaging unless the Government puts the right policies in
place.
The government in Sweden has together with BIL Sweden, the national association for the automobile industry, that are the automakers in Sweden started the work to end oil dependency. One-fifth of cars in Stockholm can run on alternative fuels, mostly ethanol fuel. Also Stockholm will introduce a fleet of Swedish-made hybrid ethanol-electric buses. In 2005, oil phase-out in Sweden by 2020 was
announced.
Biofuels in other countries
In China, the government is making E10 blends mandatory in five provinces that account for 16% of the nation's passenger cars. In Southeast Asia, Thailand has mandated an ambitious 10% ethanol mix in gasoline starting in 2007. For similar reasons, the palm oil industry plans to supply an increasing portion of national diesel fuel requirements in Malaysia and
Indonesia. In Canada, the government aims for 45% of the country’s gasoline consumption to contain 10% ethanol by 2010. Colombia mandates the use of 10% ethanol in all gasoline sold in cities with populations exceeding
500,000. In Venezuela, the state oil company is supporting the construction of 15 sugar cane distilleries over the next five years, as the government introduces a E10 (10% ethanol) blending
mandate.
In India, a bioethanol program calls for E5 blends throughout most of the country targeting to raise this requirement to E10 and then E20.
Biofuels in developing countries
Biofuel industries are becoming established in many developing countries. Many developing countries have extensive biomass resources that are becoming more valuable as demand for biomass and biofuels increases. The approaches to biofuel development in different parts of the world varies. Countries such as India and China are developing both bioethanol and biodiesel programs. India is extending plantations of jatropha, an oil-producing tree that is used in biodiesel production. The Indian sugar ethanol program sets a target of 5% bioethanol incorporation into transport
fuel. China is a major bioethanol producer and aims to incorporate 15% bioethanol into transport fuels by 2010. Costs of biofuel promotion programs can be very high,
though.
Amongst rural populations in developing countries, biomass provides the majority of fuel for heat and cooking. Wood, animal dung and crop residues are commonly burned. Figures from the International Energy Agency show that biomass energy provides around 30% of the total primary energy supply in developing countries; over 2 billion people depend on biomass fuels as their primary energy
source.
The use of biomass fuels for cooking indoors is a source of health problems and pollution. 1.3 million deaths were attributed to the use of biomass fuels with inadequate ventilation by the International Energy Agency in its World Energy Outlook 2006. Proposed solutions include improved stoves and alternative fuels. However, fuels are easily damaged, and alternative fuels tend to be expensive. People in developing countries are unlikely to be able to afford to put these solutions in place. Organizations such as Intermediate Technology Development Group work to make improved facilities for biofuel use and better alternatives accessible to those who cannot get them.
Current issues in biofuel production and use
Biofuels are proposed as having such benefits as: reduction of greenhouse gas emissions, reduction of fossil fuel use, increased national energy security, increased rural development and a sustainable fuel supply for the future.
However, biofuels are also seen as having limitations. The feedstocks for biofuel production must be replaced rapidly and biofuel production processes must be designed and implemented so as to supply the maximum amount of fuel at the cheapest cost, while providing maximum environmental benefits. Broadly speaking, first generation biofuel production processes cannot supply us with more than a few percent of our energy requirements sustainably. The reasons for this are described below. Second generation processes can supply us with more biofuel, with better environmental gains. The major barrier to the development of second generation biofuel processes is their capital cost: establishing second generation biodiesel plants has been estimated at
€500million.
Carbon emissions
Biofuels and other forms of renewable energy aim to be carbon neutral or even carbon negative. Carbon neutral means that the carbon released during the use of the fuel, e.g. through burning to power transport or generate electricity, is reabsorbed and balanced by the carbon absorbed by new plant growth. These plants are then harvested to make the next batch of fuel. Carbon neutral fuels lead to no net increases in human contributions to atmospheric carbon dioxide levels, reducing the human contributions to global warming. A carbon negative aim is achieved when a portion of the biomass is used for carbon
sequestration.
In practice, biofuels are neither carbon neutral or carbon negative. This is because energy is required to grow crops and process them into fuel. Examples of energy use during the production of biofuels include: fertilizer manufacture, fuel used to power machinery, and fuel used to transport crops and fuels to and from biofuel processing plants. The amount of fuel used during biofuel production has a large impact on the overall greenhouse gas emissions savings achieved by biofuels.
A 2007 study by scientists from Britain, U.S., Germany, Switzerland and including Professor Paul Crutzen, who won a Nobel Prize for his work on ozone, have reported that measurements of emissions from the burning of biofuels derived from rapeseed and corn have been found to produce more greenhouse gas emissions than they
save. The advantages of reduced carbon dioxide emissions are more than offset by increased nitrous oxide emissions. Nitrous oxide is both a potent greenhouse gas and a destroyer of atmospheric ozone.
The claim that biofuels result in emissions savings has also been critiqued on the grounds that it overlooks the 'displacement' effects of large-scale biofuel production, in terms of its direct and indirect role in promoting land use changes and soil carbon
losses.
The carbon emissions (Carbon footprint) produced by biofuels are calculated using a technique called Life Cycle Analysis (LCA). This uses a "cradle to grave" or "well to wheels" approach to calculate the total amount of carbon dioxide and other greenhouse gases emitted during biofuel production, from putting seed in the ground to using the fuel in cars and trucks. Many different LCAs have been done for different biofuels, with widely differing results. The majority of LCA studies show that biofuels provide significant greenhouse gas emissions savings when compared to fossil fuels such as petroleum and diesel.[citation needed] Therefore, using biofuels to replace a proportion of the fossil fuels that are burned for transportation can reduce overall greenhouse gas emissions.
The well-to-wheel analysis for biofuels has shown that first generation biofuels can save up to 60% carbon emission and second generation biofuels can save up to 80% as opposed to using fossil fuels.[44] However these studies do not take into account emissions from nitrogen fixation, deforestation, land use, or any indirect emissions.
This does assume however that the land used for growing the crops would alternatively be desert or paved area. If the land was previously a (tropical rain-) forest, the carbon absorption of this forest should be deducted from the greenhouse gas savings. This implies that the net effect of burning bio-fuels is an increase in greenhouse gasses. This effect should be incorporated in the LCA, to get a proper overview of the total net effect. Using waste material from plantation forests on previous agricultural land could be carbon positive, due to the carbon stored below ground in the root systems.
A 2008 study conducted by the University of Minnesota[45] found that:
...converting rainforests, peatlands, savannas, or grasslands to produce food-based biofuels in Brazil, Southeast Asia, and the United States creates a ‘biofuel carbon debt’ by releasing 17 to 420 times more CO2 than the annual greenhouse gas (GHG) reductions these biofuels provide by displacing fossil fuels.
The study not only takes into account removal of the original vegetation (as timber or by burning) but also the biomass present in the soil, for example roots, which is released on continued plowing. Another 2008 study by Princeton
University reached similar conclusions and found that:
...corn-based ethanol, instead of producing a 20% savings, nearly doubles greenhouse emissions over 30 years and increases greenhouse gases for 167 years.
Rising food prices — the "food vs. fuel" debate
This topic is internationally controversial, with good-and-valid arguments on both sides of the onging debate. Prices on a number of food types used for biofuel have doubled in the last couple years. There are those that say biofuel is not the main cause. Some say the problem is a result of government actions to support biofuels. Others say it is just due to oil price increases. The impact of food price increases is greatest on poorer countries.[47] Some have called for a freeze on biofuels. Some have called for more funding of second generation biofuels which should not compete with food production so
much.
Sustainability of Ethanol production
Ethanol fuel production consumes large quantities of unsustainable petroleum and natural gas. Even with the most-optimistic energy return on investment claims, in order to use 100% solar energy to grow corn and produce ethanol (fueling farm-and-transportation machinery with ethanol, distilling with heat from burning crop residues, using no fossil fuels), the consumption of ethanol fuel to replace current U.S. petroleum use alone would require about 75% of all cultivated land on the face of the Earth, with no ethanol available for other countries, or sufficient food and water for humans and animals. Scientists at the Paris Global Science Forum Conference on Scientific Challenges for Energy Research believe that many biofuels are therefore non-scalable non-solutions to our current worldwide energy
crisis.
Soil Erosion, Deforestation, and Biodiversity
It is important to note that carbon compounds in waste biomass that is left on the ground are consumed by other microorganisms. They break down biomass in the soil to produce valuable nutrients that are necessary for future crops. On a larger scale, plant biomass waste provides small wildlife habitat, which in turn ripples up through the food chain. The widespread human use of biomass (which would normally compost the field) would threaten these organisms and natural habitats. When cellulosic ethanol is produced from feedstock like switchgrass and saw grass, the nutrients that were required to grow the lignocellulose are removed and cannot be processed by microorganisms to replenish the soil nutrients. The soil is then of poorer quality. Loss of ground cover root structures accelerates unsustainable soil
erosion.
Significant areas of native Amazon rainforest have been cleared by slash and burn techniques to make room for sugar cane production, which is used in large part for ethanol fuel in Brazil, and growing ethanol exports. Large-scale deforestation of mature trees (which help remove CO2 through photosynthesis — much better than does sugar cane or most other biofuel feedstock crops do) contributes to un-sustainable global warming atmospheric greenhouse gas levels, loss of habitat, and a reduction of valuable
biodiversity.
A portion of the biomass should be retained onsite to support the soil resource. Normally this will be in the form of raw biomass, but processed biomass is also an option. If the exported biomass is used to produce syngas, the process can be used to co-produce biochar, a low-temperature charcoal used as a soil amendment to increase soil organic matter to a degree not practical with less recalcitrant forms of organic carbon. For co-production of biochar to be widely adopted, the soil amendment and carbon sequestration value of co-produced charcoal must exceed its net value as a source of
energy.
Impact on water resources
Increased use of biofuels puts increasing pressure on water resources in at least two ways: water use for the irrigation of crops used as feedstocks for biodiesel production; and water use in the production of biofuels in refineries, mostly for boiling and cooling.
Irrigation of feedstocks
The amount of water needed to grow feedstock depends on the amount of rainfall. In areas with sufficient rainfall feedstocks can be grown without any irrigation, such as corn or sugarcane in areas with sufficient rainfall and Jatropha, which is only cultivated under rainfed conditions.
However, in many parts of the world supplemental or full irrigation is needed to grow feedstocks. For example, if in the production of corn (maize) half the water needs of crops are met through irrigation and the other half through rainfall, about 860 liters of water are needed to produce one liter of ethanol.[54] This is 215 times more than the amount of water per liter of ethanol used in the refining process.
Water use in refineries
Using the example of ethanol, a typical ethanol factory producing 50m gallons of biofuels a year needs about 500 gallons of water a minute, corresponding to about 4 gallons of water per gallon of biofuel. Ethanol plants now use about half as much water per gallon of ethanol as they did a decade ago.
In the United States, the number of ethanol factories has almost tripled from 50 in 2000 to about 140 in 2008. A further 60 or so are under construction, and many more are planned. Projects are being challenged by residents at courts in Missouri (where water is drawn from the Ozark Aquifer), Iowa, Nebraska, Kansas (all of which draw water from the non-renewable Ogallala Aquifer), central Illinois (where water is drawn from the Mahomet Aquifer) and Minnesota.[55] The Energy Independence and Security Act of 2007 aims at increasing biofuels production in the U.S. to 36 billion gallons per year by 2022.
Using the ratio of 4 gallons of water per gallon of ethanol mentioned above this would require about 144 billion gallons of water (554 million cubic meters) per year for refining, corresponding to 4 percent of current depletion of the Ogallala aquifer, the largest aquifer in the United States. While water use for refining of biofuel can have significant local impacts, its overall impact on the water balance of a larger region thus seems to be relatively limited.
Human Rights Abuses
In some locations such as Indonesia deforestation for Palm Oil plantations is leading to displacement of Indigenous peoples. Also, extensive use of pesticide for biofuel crops is reducing clean water
supplies.
Environmental organizations stance
Some mainstream environmental groups support biofuels as a significant step toward slowing or stopping global climate change.[citation needed] However, biofuel production can threaten the environment if it is not done sustainably. This finding has been backed by reports of the UN,[56] the IPCC,[57] and some other smaller environmental and social groups as the EEB[58] and the Bank Sarasin,[59] which generally remain negative about biofuels.
As a result, governmental and environmental organisations are turning against biofuels made at a non-sustainable way (hereby preferring certain oil sources as jatropha and lignocellulose over palm
oil) and are asking for global support for this.
Also, besides supporting these more sustainable biofuels, environmental organisations are redirecting to new technologies that do not use internal combustion engines such as hydrogen and compressed
air.
Biofuels produce greenhouse gas emissions during their manufacture. The source of these emissions are: fertilisers and agricultural processing, transportation of the biomass, processing of the fuels, and transport and delivery of biofuels to the consumer. Some biofuel production processes produce far fewer emissions than others; for example sugar cane cultivation requires fewer fertiliser inputs than corn cultivation, therefore sugar cane bioethanol reduces greenhouse gas emissions more effectively than corn derived bioethanol. However, given the appropriate agricultural techniques and processing strategies, biofuels can provide emissions savings of at least 50% when compared to fossil fuels such as diesel and petroleum.
The increased manufacture of biofuels will require increasing land areas to be used for agriculture. Second generation biofuel processes can ease the pressure on land, because they can use waste biomass, and existing (untapped) sources of biomass such as crop residues and potentially even marine algae.
In some regions of the world, a combination of increasing demand for food, and increasing demand for biofuel, is causing deforestation and threats to biodiversity. The best reported example of this is the expansion of oil palm plantations in Malaysia and Indonesia, where rainforest is being destroyed to establish new oil palm plantations. It is an important fact that 90% of the palm oil produced in Malaysia is used by the food
industry; therefore biofuels cannot be held solely responsible for this deforestation. There is a pressing need for sustainable palm oil production for the food and fuel industries; palm oil is used in a wide variety of food products. The Roundtable on Sustainable Biofuels is working to define criteria, standards and processes to promote sustainably produced
biofuels. Palm oil is also used in the manufacture of detergents, and in electricity and heat generation both in Asia and around the world (the UK burns palm oil in coal-fired power stations to generate electricity).
Significant area is likely to be dedicated to sugar cane in future years as demand for ethanol increases worldwide. The expansion of sugar cane plantations will place pressure on environmentally-sensitive native ecosystems including rainforest in South
America. In forest ecosystems, these effects themselves will undermine the climate benefits of alternative fuels, in addition to representing a major threat to global
biodiversity.
Although biofuels are generally considered to improve net carbon output, biodiesel and other fuels do produce local air pollution, including nitrogen oxides, the principal cause of smog.[citation needed]
Potential for poverty reduction
Researchers at the Overseas Development Institute have argued that biofuels could help to reduce poverty in the developing world, through increased employment, wider economic growth multipliers and energy price effects. However, this potential is described as 'fragile', and is reduced where feedstock production tends to be large scale, or causes pressure on limited agricultural resources: capital investment, land, water, and the net cost of food for the poor.
With regards to the potential for poverty reduction or exacerbation, biofuels rely on many of the same policy, regulatory or investment shortcomings that impede agriculture as a route to poverty reduction. Since many of these shortcomings require policy improvements at a country level rather than a global one, they argue for a country-by-country analysis of the potential poverty impacts of biofuels. This would consider, among other things, land administration systems, market coordination and prioritising investment in biodiesel, as this 'generates more labour, has lower transportation costs and uses simpler
technology'.
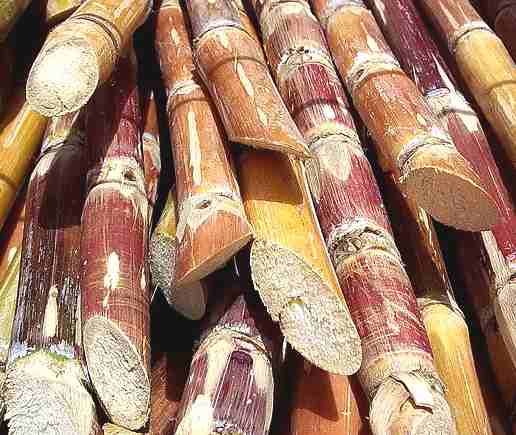
Sugar
cane cut stems
Biofuel prices
Retail, at the pump prices, including U.S. subsidies, Federal and state motor taxes, B2/B5 prices for low-level Biodiesel (B2-B5) are lower than petroleum diesel by about 12 cents, and B20 blends are the same per unit of volume as
petrodiesel.
Due to the 1/3 lower energy content of ethanol fuel, even the heavily-subsidized net cost to drive a specific distance in flexible-fuel vehicles is higher than current gasoline prices.
Energy efficiency and energy balance of biofuels
Production of biofuels from raw materials requires energy (for farming, transport and conversion to final product, and the production / application of fertilizers, pesticides, herbicides, and fungicides). We cannot continue stripping ecosystems of large quantities of biomass year-after-year, without environmental
consequences.
The level of energy expenditure varies by location: more-intensive, high-yield, genetically-engineered crop agricultural regimes such, as those found in Western countries, are more energy and irrigation intensive. The more machinery is used for farming, the greater the energy expended in the process; developing countries tend to have less-energy-intensive, lower-yield, agricultural methods.
It is possible to produce biomass without incurring large agricultural energy costs: for example, wild-harvesting grass and wood byproducts from established forests can be done without much energy input. However, the yield of biomass from such resources is not consistent or large enough to support biofuel manufacture on a large scale. Producing biofuels from cellulose is more energy intensive than from sugar and starch foods, like corn and soy, which negatively impact food supply.
The energy balance of a biofuel is determined by the amount of energy put into the manufacture of fuel compared to the amount of energy released when it is burned in a vehicle. This varies by feedstock and according to the assumptions used. Biodiesel made from sunflowers may produce only 0.46 times the input rate of fuel
energy. Biodiesel made from soybeans may produce 3.2 times the input rate of fossil
fuels. This compares to 0.805 for gasoline and 0.843 for diesel made from
petroleum. Biofuels may require higher energy input per unit of BTU energy content produced than fossil fuels: petroleum can be pumped out of the ground and processed more efficiently than biofuels can be grown and processed. However, this is not necessarily a reason to use oil instead of biofuels, nor does it have an impact on the environmental benefits provided by a given biofuel.
Other factors connected to energy balance include a) cost and b) environmental impact. High energy impacts do not necessarily mean that the resulting fuel will be bad for the environment: energy can be derived from renewable resources to power biofuel manufacture.
Energy balance is not necessarily a measure of a good biofuel. Biofuels must eventually become cost-effective without heavy subsidies: affordable, lower total cost per unit of distance, environmentally sustainable, abundant, and provide lower greenhouse gas (GHG) emissions (all things considered) than fossil fuels. As fossil fuel peak oil supply declines, prices increase, and biofuel technology (like collocation) improves, progress toward these challenging goals should slowly improve.
Energy balance/ efficiency of conversion is relevant when considering how best to use a given amount of biomass resources. For example, given limited resources should biomass be converted into heat and power or liquid transport fuels? Looking at energy balance and the efficiency of energy conversion can help to use biomass resources efficiently and with maximum environmental gain.
Studies have been done that calculate energy balances for biofuel production. Some of these show large differences depending on the biomass feedstock used and
location.
To explain one specific example, a June 17, 2006 editorial in the Wall. St. Journal stated, "The most widely cited research on this subject comes from Cornell's David Pimental and Berkeley's Ted Patzek. They've found that it takes more than a gallon of fossil fuel to make one gallon of ethanol — 29% more. That's because it takes enormous amounts of fossil-fuel energy to grow corn (using fertilizer and irrigation), to transport the crops and then to turn that corn into
ethanol."
The energy balance is more favourable for biofuels made from crops grown in subtropical or tropical areas than those made from crops grown in temperate areas, where there is snow on the ground part of the year, and the growing season is short. This is largely due to the increased yield of biomass from crops in areas that receive more annual sunlight, with a year-round growing season (as in equatorial Brazil).
Life cycle assessments of biofuel production show that under certain circumstances, biofuels produce only limited savings in energy and greenhouse gas emissions. Fertiliser inputs and transportation of biomass across large distances can reduce the GHG savings achieved. The location of biofuel processing plants can be planned to minimize the need for transport, and agricultural regimes can be developed to limit the amount of fertiliser used for biomass production. A European study on the greenhouse gas emissions found that well-to-wheel (WTW) CO2 emissions of biodiesel from seed crops such as rapeseed could be almost as high as fossil diesel. It showed a similar result for bio-ethanol from starch crops, which could have almost as many WTW CO2 emissions as fossil petrol. This study showed that second generation biofuels have far lower WTW CO2
emissions.
Other independent LCA studies show that biofuels save around 50% of the CO2 emissions of the equivalent fossil fuels. This can be increased to 80-90% GHG emissions savings if second generation processes or reduced fertiliser growing regimes are used. Further GHG savings can be achieved by using by-products to provide heat, such as using bagasse to power ethanol production from
sugarcane.
Collocation of synergistic processing plants can enhance efficiency. One example is to use the exhaust heat from an industrial process for ethanol production, which can then recycle cooler processing water, instead of evaporating hot water that warms the
atmosphere.
Biofuels and solar energy efficiency
Biofuels from plant materials convert energy that was originally captured from solar energy via photosynthesis. A comparison of conversion efficiency from solar to usable energy (taking into account the whole energy budgets) shows that
photovoltaics are 100 times more efficient than corn
ethanol and 10 times more efficient than the best biofuel.
LINKS
and REFERENCE
-
SmartWay
Grow & Go.
-
N2O
release from agro-biofuel production negates global warming reduction
by replacing fossil fuels.
-
Biofuels
Deemed a Greenhouse Threat.
-
Biofuel
demand leading to human rights abuses, report claims Jessica
Aldred, guardian.co.uk
-
freetheplant.net
-
National
Geographic, Green Dreams, Oct 2007
-
European
Environment Agency (2006) How much bioenergy can Europe produce
without harming the environment? EEA Report no. 7
-
Marshall,
A. T. (2007) Bioenergy from Waste: A Growing Source of Power, Waste
Management World Magazine, April, p34-37
-
IPCC
Third Assessment Report, accessed August 31, 2007.
-
Non-CO2
Gases Economic Analysis and Inventory: Global Warming Potentials and
Atmospheric Lifetimes, U.S. Environmental Protection Agency,
accessed August 31, 2007
-
Frank
Keppler, John T. G. Hamilton, Marc Bra, and Thomas Röckmann (2006).
"Methane emissions from terrestrial plants under aerobic
conditions". Nature 439: 187-191. doi:10.1038/nature04420.
-
UN
biofuels report
-
http://www.biodiesel.de/
-
http://www.biodieselfillingstations.co.uk
-
http://www.butanol.com/
-
Andrew
Bounds (September 10 2007). "OECD
warns against biofuels subsidies". Financial
Times.
-
With
only 2/3 the energy of gasoline, ethanol costs more per mile.
zFacts.com (27 Apr 2007).
-
http://www.hydrogensolar.com/
-
greenfuelonline.com
-
http://www.renewable-energy-world.com/
-
Chris
Somerville. "Development
of Cellulosic Biofuels" (PDF).
U.S.
Dept. of Agriculture.
-
Eviana
Hartman (January 6, 2008). "A
Promising Oil Alternative: Algae Energy". Washington
Post.
-
Globeco
biodegradable bio-diesel
-
Friends
of Ethanol.com biodegradable ethanol
-
Low
Cost Algae Production System Introduced
-
IEA
bioenergy
-
Press
Conference Launching International Biofuels Forum. United
Nations Department of Public Information (2 March 2007).
-
G.M.
Buys Stake in Ethanol Made From Waste By MATTHEW L. WALD
Published: January 14, 2008 New
York Times Link
-
Bush
Signs Energy Independence and Security Act of 2007.
-
Food
Prices: Cheap No More.
-
Roger
Harrabin (14 January 2008). "EU
rethinks biofuels guidelines". BBC
News.
-
EU
biofuels barometer: Germany & France in the lead (July 30,
2007).
-
Richard
Black (14 January 2008). "Biofuels
'are not a magic bullet'". BBC
News.
-
"Sustainable
biofuels: prospects and challenges". The Royal Society (14
Jan 2008).
-
Prime
Minister's Office Commission on Oil Independence. Making
Sweden an OIL-FREE Society
-
Press
release from the Presidencia De La República de Colombia "COLOMBIA
SE ALISTA PARA ENTRAR A LA ERA DEL ETANOL"
-
Ethanol
India website
-
See
Jörg Peters and Sascha Thielmann (2008) Promoting Biofuels:
Implications for Developing Countries, Ruhr Economic Papers #38 ([www.rwi-essen.de]
for download)
-
world
resources institute document on wood fuels (PDF)
-
Nexant
Chem Systems study
-
[1]
“Carbon negative energy to reverse global warming” (a posting to
Energy Resources Group on Yahoo). Report on the symposium (EACU) in
2004 at the University of Georgia at Athens (Georgia, USA). Several
scientists from very diverse disciplins: chemistry, archeology,
physics, anthropology, microbiology, pedology, agronomy, researchers
in renewable energies, and representatives for the DOE (Department of
Environment), USDA and industry. Aim: to observe the evidences of
massive utilisations of carbon in history, make a synopsis on present
research, and study how carbon-negative energy can be economically
deployed today” (See also [2])
-
Smith,
Lewis (The Times) (2007, Sept.). "Study:
Biofuels May Produce More Greenhouse Gas Than Oil" (HTML).
-
Paving
the way for Agrofuels: EU policy, sustainability criteria, and climate
calculations
-
Concawe
European WTW study
-
Land
Clearing and the Biofuel Carbon Debt Joseph Fargione, Jason Hill,
David Tilman, Stephen Polasky, Peter Hawthorne Published Online
February 7, 2008 Science doi:10.1126/science.1152747
-
Use
of U.S. Croplands for Biofuels Increases Greenhouse Gases Through
Emissions from Land Use Change Timothy Searchinger, Ralph
Heimlich, R. A. Houghton, Fengxia Dong, Amani Elobeid, Jacinto Fabiosa,
Simla Tokgoz, Dermot Hayes, Tun-Hsiang Yu Published Online February 7,
2008 Science doi:10.1126/science.1151861
-
"NGO
has biofuel concerns". BBC
News (01 November, 2007).
-
Green
Dreams J.K. Bourne JR, R. Clark, National
Geographic Magazine, October 2007 p. 41, Article
-
The
Economist – The End Of Cheap Food.
-
Global
Science Forum Conference on Scientific Challenges for Energy Research:
Energy At The Crossroads.
-
National
Soil Erosion Research Laboratory. U.S. Department of Agriculture
(03/05/2008).
-
www.journals.royalsoc.ac.uk
“Prehistorically modified soils of central Amazonia:
a model for sustainable agriculture in the twenty-first
century”, by Bruno Glaser at the Institute of Soil Science and Soil
Geography, University of Bayreuth (see the “Terra
Preta Web Site”). Extract available here.
Published online December 20, 2006 in Philosophic Transactions Royal
Society B (2007) 362, 187–196. doi:10.1098/rstb.2006. 1978. This
article studies the evidences concerning the process of generation of
Terra preta as well as the reasons why its organic matter's and
nutrients' retention is so superior to the surrounding soils.
-
To
calculate this relationship, one has to take into account that
irrigated corn needs about 560 cubic meters (2.1m gallons) of water
per ton of corn (as quoted in[Eco-World.
Ed Ring:Is bio-fuel water positive? June 4th, 2007] using
estimates from the University of Colorado and UNESCO, as well as a
clarification by David Nielsen, Research Agronomist, USDA-ARS, Akron,
Colorado, posted on July 19, 2007.) A good ethanol yield is about 480
galons per acre per year, and a typical corn yield is 5.6 tons per
acre per year. Assuming that half the crop water needs can be met
through rainfall, this would mean that still 1,570 cubic meter (1.57m
liter) - 280 cubic meter of water per ton, multiplied by 5.6 tons per
acre - of irrigation water are needed per acre per year to produce
1,817 liter (480 galons) of ethanol.
-
The
Economist, March 1st 2008, Ethanol and water: don't mix, p. 36
-
U.N.
raises possible negative impact of biofuels on environment, food
security.
-
IPCC's
Mitigation of Climate Change report negative on biofuels.
-
Biofuels
no panacea (PDF).
-
Biofuels
— Transporting Us to a Fossil-Free Future?.
-
Governmental
(OECD) organisations against unsustainable biofuels.
-
Friends
of the Earth, Oxfam, ... preferring jatropha over palm oil.
-
Environmental
organisations against non-sustainable biofuels 1.
-
Environmental
organisations against non-sustainable biofuels 2.
-
Zero
Carbon Environmental Organisation.
-
Malaysian
Palm Oil Council.
-
Roundtable
on Sustainable Biofuels website.
-
BBC
News.
-
Agrofuels
— towards a reality check in nine key areas.
-
Biofuels,
Agriculture and Poverty Reduction. Overseas Development Institute
(2007).
-
"Clean
Cities Alternative Fuel Price Report" (PDF). U.S. Dept. of
Energy (July 2007).
-
Cellulosic
ethanol will not save us
-
Pimentel,
D.; T.W. Patzek (2005). "Ethanol
Production Using Corn, Switchgrass, and Wood; Biodiesel Production
Using Soybean and Sunflower". Natural Resources Research
14 (1): 65-75. doi:10.1007/s11053-005-4679-8.
-
John
Sheehan; Vince Camobreco, J. Duffield, M. Graboski, H. Shapouri (May
1998). Life
Cycle Inventory of Biodiesel and Petroleum Diesel (PDF),
National Renewable Energy Laboratory. NREL/SR-580-24089.
-
Shapouri
(2002), The
Energy Balance of Corn Ethanol: An Update, USDA,
Agricultural Economic Report No. 813, <http://www.ethanolrfa.org/objects/documents/79/aer-813.pdf>.
Retrieved on 25 January 2008(see
page 8)
-
"Biofuel"
does not necessarily mean ecologically friendly (EMPA report May
2007).
-
An
Energy Field of Dreams The Wall St. Journal, June 17, 2006
-
European
VIEWLS Biofuel report p.28 fig.4 (PDF).
-
Concawe
Well to Wheels LCA for biofuels.
-
"FPL
Energy finds partner for citrus-peel-to-ethanol plant". Biomass
Magazine (October 2007).
-
Markman,
Jon, "Shuck the ethanol and let solar shine" 10/11/2007
-
"Biofuel
vs. Photovoltaics" EcoWorld

New
energy drinks for performers
..
Thirst for Life

330ml
Earth can - the World in Your Hands
|